NEUROPAIN develops physiological, pre-clinical and clinical research on pain. It develops its research working
almost exclusively on human beings
The group is committed with three main research axes:
- the study of cortical mechanisms leading to, and modulating pain perception in humans (Pain Physiology);
- the use of neurophysiological techniques to improve our understanding, diagnosis and prediction of neuropathic pain (PathoPhysiology of Pain);
- the improvement of cortical neurostimulation procedures for pain relief through a better comprehension of their mechanisms (NeuroStimulation).
The team is physically located within the Neurological Hospital of Lyon and the University hospital of Saint-Etienne. Most of the team members have clinical activities, hence ensuring direct access to clinical facilities and patient wards. NeuroPain has dedicated systems for high-density electrophysiology (2x128- channel EEG) and cortical stimulation (rTMS, tDCS, epidural and intracortical stimulation), as well as 3D neuronavigation facilities with robotized arm and several multi-modal sensory stimulation systems, including two nociceptive-specific Nd:YAP laser stimulators. We have access to MEG, fMRI and PET- scan facilities, as well as to the platform for intracranial EEG, which is coordinated by one of our team members. NeuroPain is also founding member of, and directly involved in the development of the platform “Neuro-Immersion”, coupling Virtual Reality with HD-EEG, TMS and 3-D real-time kinematics.
NeuroPain joins efforts of 20 tenured members: neurobiologists, neurologists, psychologists, neurosurgeons, research engineers.
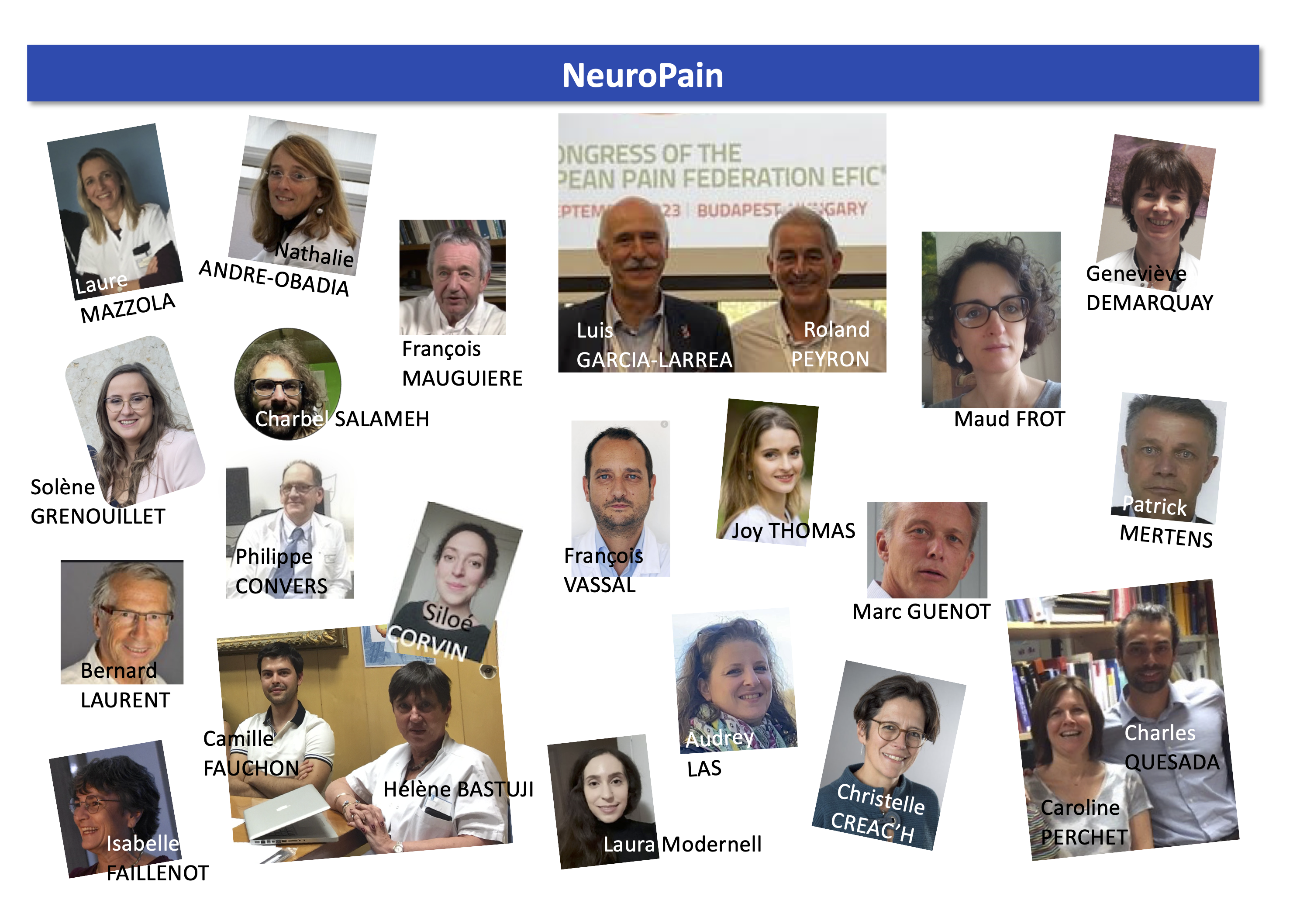
- 3 researchers INSERM or University Lyon1
- 3 technical / ingeneer support staff
- 13 clinicians (including 2 with 50% secured research time)
Psychophysics, EEG, intracranial EEG, anatomical and functional MRI, PET, neuronavigated rTMS, tDCS
Signal and image processing, brain oscillations, source modeling, connectivity.
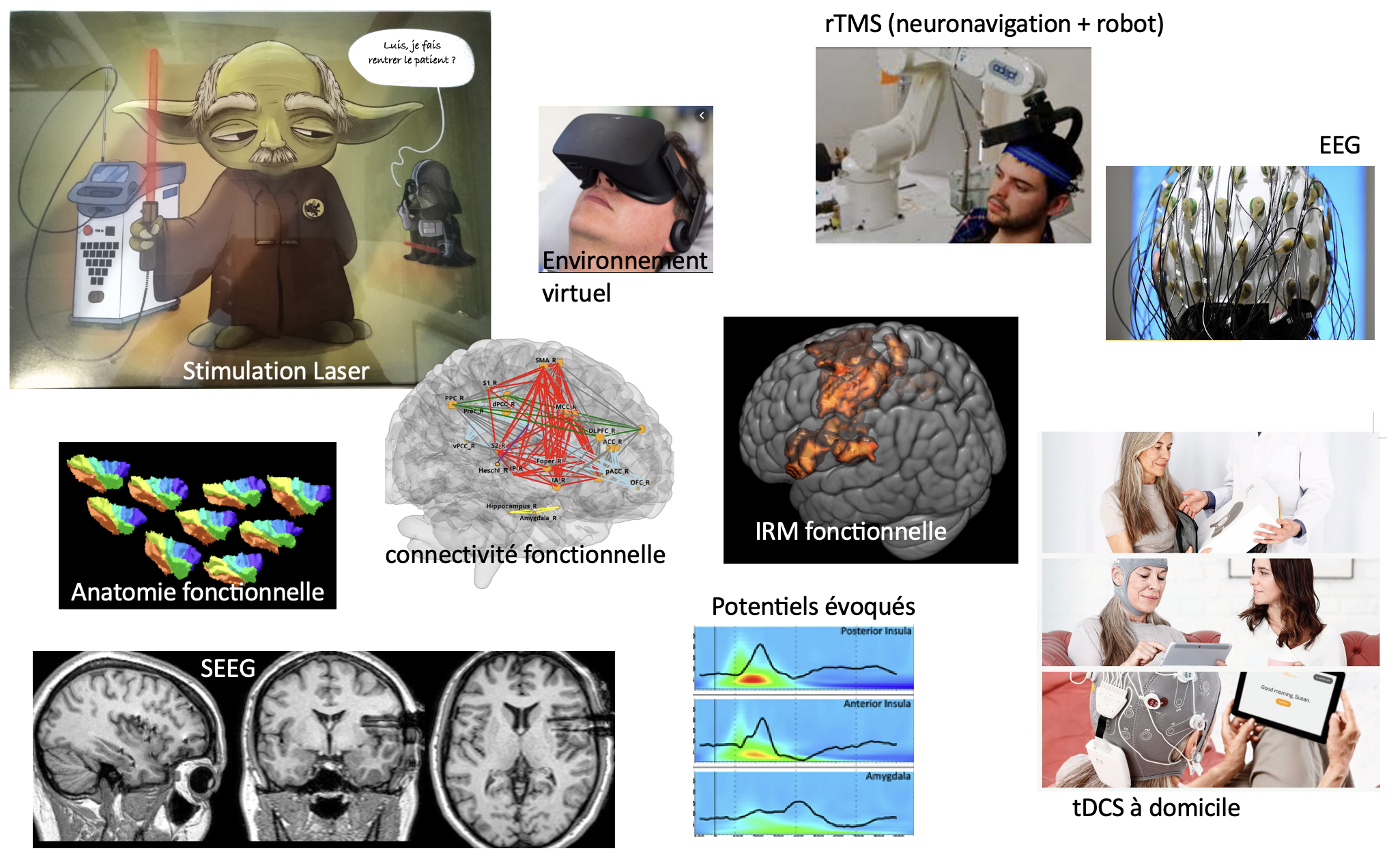
Physiology and Pain modulation
• Analyse spatio-temporelle du traitement de l’information douloureuse
- Compréhension des déterminants corticaux de la perception subjective de la douleur
(M. Frot, M. Guenot , H. Bastuji)
- Analyses des interactions fonctionnelles intra-cérébrales en lien avec la perception douloureuse
(C. Fauchon, L. Modernell, I. Faillenot)
• Interaction avec des mécanismes cognitifs attentionnels et émotionnels
- Intégration corticale des visages émotionnels douloureux
(M. Frot)
- Décodage des signaux douloureux dans un stimulus auditif, le cri du bébé
(S. Corvin, C. Fauchon, I. Faillenot, R. Peyron, Coll N. Mathevon, ENES)
• Mémorisation de la douleur
(M. Frot, C. Perchet)
- Mémorisation de la douleur en lien avec un contexte
- Mémorisation du contexte associé à la douleur
Diagnostic, prévention et caractérisation de la douleur neuropathique
• Projet européen sur la détection des biomarqueurs de la douleur
(L. Garcia-Larrea)
• Potentiels Évoqués Laser en clinique pour le diagnostic des douleurs neuropathiques
(L. Garcia-Larrea, C. Perchet)
Traitements non médicamenteux de la douleur chronique
• Etude de la rTMS et tDCS à visée antalgique
- Suivi d’une cohorte de patients traités par rTMS puis par électrode corticale
(R. Peyron, J. Thomas, C. Quesada, L. Garcia-Larrea, F. Vassal)
- Programme de stimulation électrique transcrânienne à domicile (Projet STIMADOM)
(C. Perchet, L. Garcia-Larrea)
• Couplage de la rTMS à la réalité virtuelle immersive
- Optimiser les effets de la rTMS en la couplant avec la thérapie miroir (Projet ALGOMAX)
(J. Thomas, C. Fauchon, R. Peyron)
- Connectivité fonctionnelle corticale associée à l’efficacité du traitement
(J. Thomas, C. Fauchon, I. Faillenot, R. Peyron)
• Apport de la danse-thérapie chez des adolescents (Projet ALGODANSE)
(M. Frot, C. Perchet, G. Demarquay en collaboration avec le
Centre d’Etude de la Douleur de l’Hôpital Neurologique)
-
Researchers
Jean Isnard
Amandine Rey
Michel Magnin
- Post-Doc or associate researchers
Siloé Corvin (post-Doc, France)
Camille Fauchon (post-Doc, France)
Koichi Hagiwara (associated researcher, Japan)
Philip Jakson (Visiting Professor, Canada)
Benjamin Dominguez-Trejo (Visiting Professor, Mexico)
Emile Simon (associate researcher)
Agustina Lascano (visiting neurologist, Suisse)
Stéphanie Maza (associate researcher, France)
Fabio Godinho (Post-Doc, Brazil)
Nuuti Vartiainen (Post-Doc, FInland)
Florian Chouchou (Post-Doc, France)
Sigrid Shuh-Hoffer (Post-Doc, Germany)
Valery Legrain (Post-Doc, Belgium)
Ron Kuppers (Post-Doc, Belgium)
Blanca Diaz (Post-Doc, Spain)
Jordi Pedro (Post-Doc, Spain)
Lorena Guzman (Post-Doc, Venezuela)
Aline Acosta (Post-Doc, Brazil)
Cecilia Flores (Post-Doc, Spain)
- PhD
Siloé Corvin (PhD, Neurosciences, Lyon)
Juliette Gélébart(PhD, Neurosciences, Lyon)
Argitxu Caldichoury (PhD, Neurosciences, Lyon)
Camille Fauchon (PhD, Ingénierie & santé, Saint-Etienne)
Charles Quesada (PhD, Ingénierie & santé, Saint-Etienne)
Benjamin Pommier (PhD, Ingénierie & santé, Saint-Etienne)
Christophe Nuti (PhD, Ingénierie & santé, Saint-Etienne)
Claire Bradley (PhD, Neurosciences, Lyon)
Claire Czelkala (PhD, Neurosciences, Lyon)
Lea Claude (PhD, Neurosciences, Lyon)
Anaïs Chapon (PhD, Neurosciences, Lyon)
Laure Mazolla (PhD, Ingénierie & santé, Saint-Etienne)
Florence Borgetto-Pomares (PhD, Neurosciences, Lyon)
Aurelia Poujois (PhD, Ingénierie & santé, Saint-Etienne)
Florian Chouchou (PhD, Neurosciences, Lyon)
Estelle Raffin (PhD)
Laure Peter-Derex (PhD, Neurosciences, Lyon)
Joseph Maraawi (PhD)
Mustafa Mahmutoglu (visiting PhD, Germany)
Marcos-Fortunato-de-Barrios Jr (visiting PhD, Germany)
Marina Pidal (visiting PhD, Spain)
German Prados (visiting PhD, Spain)
-
master out of Lyon or St-Etienne
Marie-Hélène Tessier (Visiting Master, Canada)
Nyamh Joyce (Master Erasmus, UK)
Ruth Kirby (Master Erasmus, UK)
Temitope Lana (Master Erasmus, UK)
Eva Masson (Master, Bordeaux)
Elisa Redavide (Master Erasmus, Italy)
Inès Jani (Master, Canada)
Martin-Schulz (Visiting Master, Germany)
Quentin Welniarz (Master, ENS Paris)
Audrey Las, gestionnaire de l'équipe NeuroPain
Isabelle Faillenot, webmaster de l'équipe NeuroPain